When Jie Yin was seeking inspiration for a new class of building materials, he turned to YouTube.
The associate professor of mechanical and aerospace engineering at North Carolina State University was looking for ideas based on the ancient Japanese art of kirigami, a version of origami where paper is cut as well as folded. Yin and his collaborators wanted to adapt this two-dimensional approach, applying the principles to produce three-dimensional materials.
During his search, Yin ran across the infinity cube, or flexicube, a toy that users can manipulate to produce a variety of shapes. The team envisioned building blocks that similarly would let them produce materials that could undergo metamorphosis. With the repeating cubes, the team could create structures that start with one fundamental architecture—but, with purposefully placed incisions, could then transform into another architecture entirely.
Because the design approach is modular, designers can assemble hundreds of blocks to develop complex, dynamic, multi-level structures, then disassemble them just as easily. The flexibility makes the materials particularly well-suited for rapidly deployable structures like mobile hospitals or emergency housing, where construction standards are less restrictive than in permanent facilities.
Tailored properties
“I started with eight cubes folded into a brick shape,” Yin said. “But by ensuring freedom of movement, they can also form a closed loop.” Eight turns out to be the magic number for the blocks—four or six provides only a limited number of shapes and 10 or 12 are difficult to control.
In use, the cubes might be made of a rigid material, like wood or plastic, and connected with hinges operated by motors. Then, engineers could program the cubes to reconfigure into the shapes needed.
Each cube initially has six sides. The cubes can be hollow to reduce weight while still offering high compressive strength without shear. By removing two opposite sides of each cube, it’s possible to provide shear in a desired direction and to lock in the angle of rotation. Then, depending on where designers place the blocks in a structure, they can tune the stiffness.
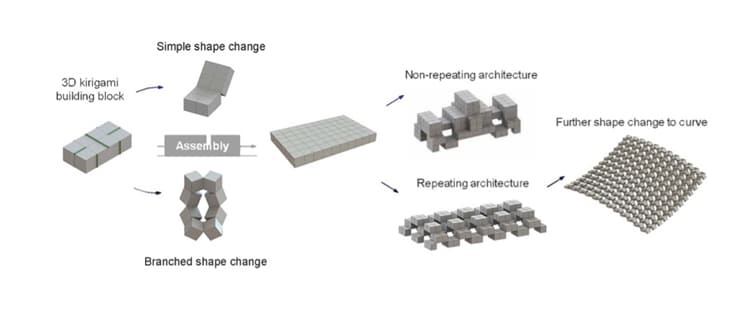
“The same blocks can be folded into a structure that can easily be compressed, then refolded into a shape that can bear a significant load,” Yin explained. “This allows you to alter the mechanical properties of a structure to perform different tasks.”
“For example, you may initially make a structure rigid,” Yin added. “But when a hurricane is coming, you can give it the flexibility to shear a bit in the wind.” The ability to select angles also allows curved surfaces, like the arched roof of the famous Sydney Opera House.
The possibilities are almost literally endless. Depending on how the cubes are connected, the building blocks can be folded into more than 300,000 different designs. But with so many options, how does a designer even get started?
Design directions
In the conventional approach, designers begin with a material or structure, then evaluate the properties. But here, the team applies inverse design. In other words, the team starts instead with the performance required, then works backward using an optimization algorithm that automates the search for a structure that provides it.
Yin and his colleagues have embarked on an ambitious plan to build a library of structure types that show what’s possible. The researchers can use data sets and train them using machine learning to determine how to put building blocks together in the optimal way to create various shapes. Once designers decide on the shape to target, they can access the steps to build it from the library. If it’s not there, they can still extrapolate from similar shapes.
Of course, it helps that the material is designed to be forgiving. “For a hospital, each cube could be a room,” Yin said. “If you don’t like your original creation, you can simply transform it to fit your needs.” When a particular structure is no longer needed, a crew can disassemble it, flatten out the building blocks into a compact form and ship them to the next location where they can be reassembled to meet new requirements.
Prototyping at scale
The team has shown the fundamental concept with a model at 1-inch scale. Next up: Moving on to a larger prototype at 1-foot dimensions to investigate real-world applications for the building blocks.
Then there’s another possibility that takes the project full circle. The team is planning to create a version as an educational toy for science, technology, engineering and mathematics (STEM) classes. The students can build structures and learn how orienting blocks in different ways impacts material properties and structure function and design.